Spring 2025 Issue Highlights
In 2024, the Shirk Athletic Center reached a new milestone with its 30th anniversary. The Center still serves as an essential community hub for campus and Bloomington-Normal.
More issue highlights
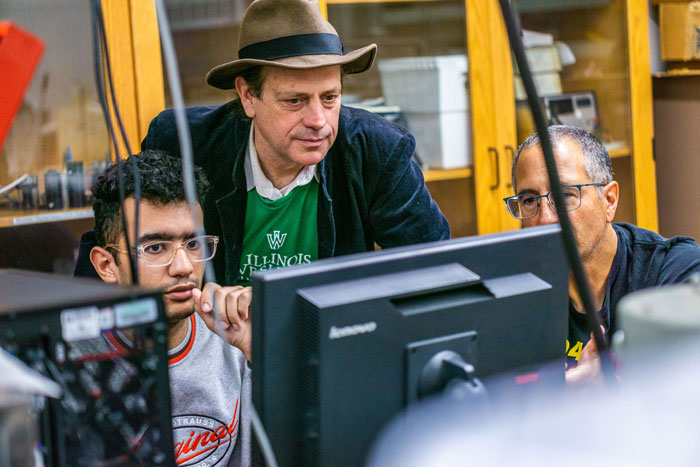
A Hearth for Haiti
Kevin Hineline ‘88 is working with IWU physics students to develop a new cooking stove for use in the most impoverished and disaster-stricken regions of the world.
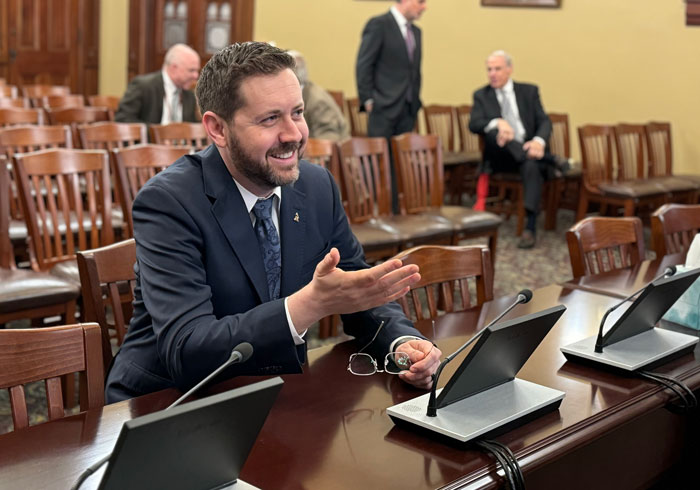
Setting a Standard
Jeff Becker ‘01 and Matt Glavin ‘01 are the minds behind Illinois' new AI regulation that's setting a standard for states across the country.
Send Us Your News
Illinois Wesleyan University Magazine wants to hear from alumni. Please share your news by using the forms listed below.
- General Alumni Updates (including address changes)
- Publications, performances, and exhibitions
- Marriage announcement
- Baby ("Tiny Titans") announcement
- Submit a digital photograph ("Titan Celebrations")
Previous issues
2025 | 2024 | 2023 | 2022 | 2021 | 2020 | 2019 | 2018 | 2017-2002
IWU Magazine staff: Chris Francis, Editor; Nick Munafo, Graphics Editor
Illinois Wesleyan University Magazine is published
by the Office of University Communications.
Content on this website copyright
of Illinois Wesleyan University.